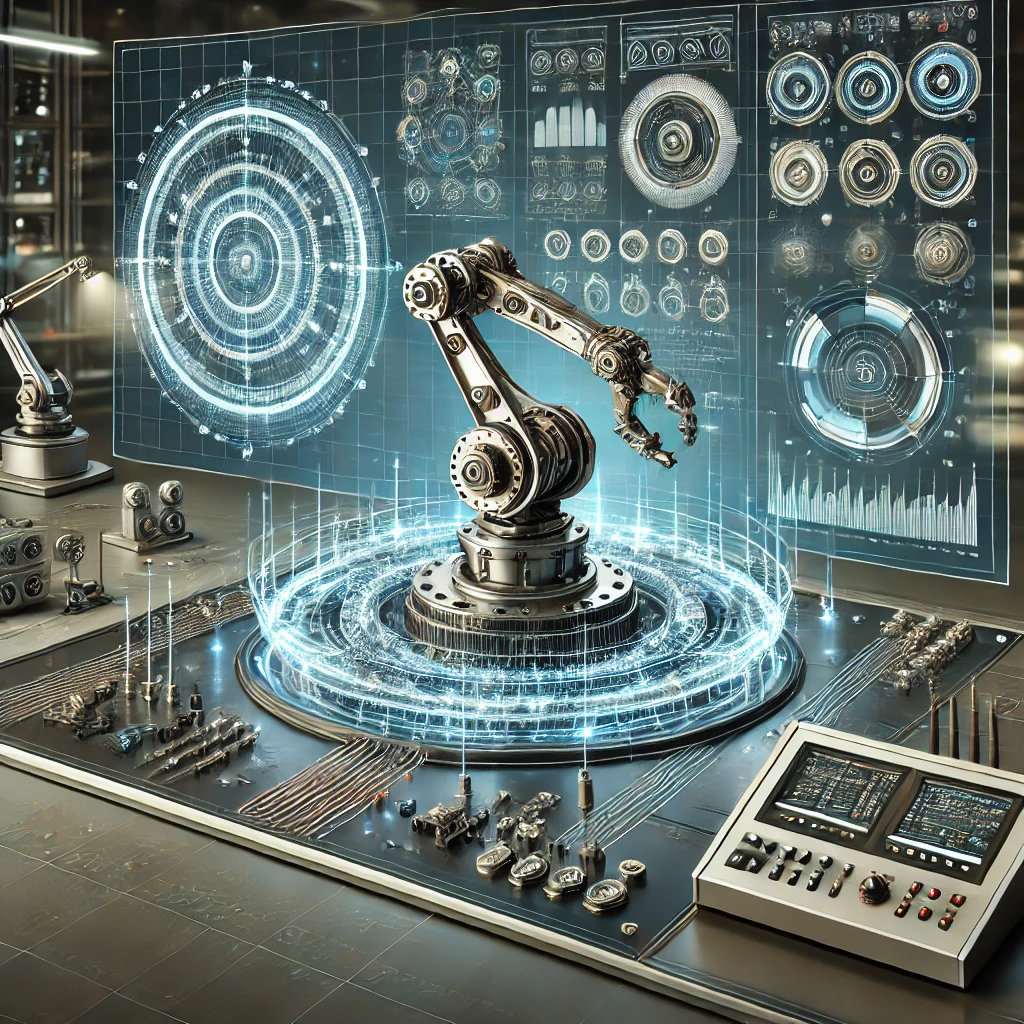
1.1) OVERVIEW AND ORIGIN OF THE CONTROL THEORY UNDER ENGINEERING AND TECHNOLOGY
Control theory is a branch of engineering and technology that studies the behavior of dynamic systems and the use of control mechanisms to achieve desired outputs through feedback and regulation. It provides a theoretical framework for designing systems that can maintain stability, enhance performance, and respond predictably to external disturbances or changes in input conditions. Control systems are critical in numerous fields, including automation, robotics, aerospace, electrical systems, and mechanical engineering.
The origin of control theory dates back to the 18th century with the invention of the centrifugal governor by James Watt, used to regulate the speed of steam engines. This device automatically adjusted the steam flow based on the engine’s speed, exemplifying one of the earliest feedback control systems. The principles behind the governor laid the foundation for modern control systems.
Significant theoretical advancements emerged in the early 20th century. Engineers like Harry Nyquist contributed to the stability analysis of feedback systems, particularly in electrical circuits. Nyquist’s stability criterion, developed in the 1930s, became a cornerstone for understanding and designing feedback systems. During the same period, Norbert Wiener introduced the concept of cybernetics, which linked control theory with communication and feedback in both mechanical systems and biological processes.
World War II marked a turning point for the field, as the need for automated systems, such as missile guidance and anti-aircraft targeting, led to the rapid development of mathematical tools for control system design. After the war, the introduction of state-space analysis by Rudolf Kalman and others in the 1960s revolutionized control engineering. Kalman’s development of the Kalman filter provided a method for optimally estimating system states, even in the presence of noise and uncertainty.
Control theory has since evolved to address increasingly complex systems, incorporating advanced computational techniques, artificial intelligence, and machine learning. Modern applications range from autonomous vehicles and smart grids to space exploration and medical systems.
For further details, references such as “Modern Control Engineering” by Ogata (2010) and “Feedback Control of Dynamic Systems” by Franklin, Powell, and Emami-Naeini (2019) provide comprehensive insights into the historical development and contemporary applications of control theory.
Control theory is a branch of engineering and mathematics that deals with the behavior of dynamic systems and their regulation using controllers. This discipline provides the tools to design systems that respond predictably to inputs and maintain stability even when subjected to external disturbances. Below is a detailed exploration of its applications across various fields in engineering and technology.
1.2.1) Electrical and Electronics Engineering
Control theory is extensively applied in designing and regulating electrical systems such as power supplies, electric drives, and communication networks. For instance, in power electronics, control techniques like pulse-width modulation (PWM) and feedback loops regulate voltage levels in power converters, ensuring stable operation under varying load conditions. In communication systems, control theory is employed in adaptive equalizers and noise cancellation systems to improve signal quality. According to Ogata (2010), control theory underpins the development of automatic voltage regulators (AVRs) used in generators to stabilize electricity supply.
1.2.2) Mechanical Engineering
In mechanical engineering, control theory is essential in robotics, mechatronics, and vehicle dynamics. Robotic systems, for example, utilize advanced control techniques to coordinate motion, balance, and precision. The PID controller, which adjusts system behavior based on proportional, integral, and derivative terms, is widely used in industrial robotics for tasks like positioning and trajectory planning. Moreover, in automotive systems, control theory facilitates anti-lock braking systems (ABS), cruise control, and stability control to enhance vehicle performance and safety (Ogata, 2010). Modern automobiles increasingly rely on model-based predictive control to optimize fuel efficiency and reduce emissions.
1.2.3) Aerospace Engineering
The aerospace industry leverages control theory in flight dynamics, navigation systems, and spacecraft attitude control. Autopilot systems use a combination of feedback and feedforward control mechanisms to maintain stable flight paths. These systems continuously monitor parameters like altitude, speed, and heading, adjusting inputs to stabilize the aircraft under varying conditions. Advanced applications include control systems for unmanned aerial vehicles (UAVs), which ensure precise maneuverability and autonomous operation (Stevens & Lewis, 2003). Similarly, in spacecraft, control theory governs thruster operation and ensures orbital stability.
1.2.4) Chemical and Process Engineering
In chemical engineering, process control ensures that industrial processes operate safely, efficiently, and within desired parameters. For example, in oil refineries, control systems regulate temperature, pressure, and chemical composition to optimize output while minimizing risks. Advanced strategies such as Model Predictive Control (MPC) use dynamic models to anticipate and correct deviations in real time, providing significant improvements in process efficiency (Seborg et al., 2010). This application is especially critical in industries where small deviations can result in hazardous conditions or substantial losses.
1.2.5) Renewable Energy Systems
Renewable energy systems, such as wind turbines and solar power plants, depend heavily on control systems to maximize energy output and maintain grid stability. For example, wind turbines use pitch control to adjust the blade angle, optimizing energy capture based on wind speed. Similarly, solar power systems employ maximum power point tracking (MPPT) algorithms to ensure the highest possible energy conversion efficiency from photovoltaic cells. Control theory also helps in integrating renewable sources into the power grid by maintaining frequency and voltage stability amidst fluctuating supply and demand (Zhu et al., 2013).
1.2.6) Biomedical Engineering
In biomedical engineering, control systems play a pivotal role in the development of medical devices like pacemakers, insulin pumps, and artificial organs. For instance, insulin pumps utilize feedback control to maintain blood glucose levels by adjusting insulin delivery based on real-time measurements. Artificial hearts and ventilators also rely on adaptive control systems to mimic natural physiological processes, ensuring patient safety and improving treatment outcomes (Astrom & Murray, 2012).
1.2.7) Autonomous Vehicles
Autonomous vehicles, including self-driving cars and drones, are among the most advanced applications of control theory. These systems integrate sensors, actuators, and control algorithms to perceive their environment, make decisions, and execute movements. For example, path-following algorithms use control theory to ensure a vehicle stays within its lane while avoiding obstacles. Additionally, advanced techniques like predictive control help optimize speed and energy efficiency in dynamic environments (Lewis et al., 2015).
1.2.7) Internet of Things (IoT)
The IoT connects devices to collect, exchange, and act on data in real-time. Control theory enables the development of smart systems that automate processes and respond dynamically to environmental changes. For instance, smart thermostats use feedback control to maintain desired temperatures, while industrial IoT applications use control systems to monitor and optimize production lines.
Control theory has proven indispensable in advancing engineering and technology by enabling the design of stable, efficient, and reliable systems. Its applications span diverse fields, from robotics and aerospace to renewable energy and biomedical devices. As technology evolves, control theory continues to drive innovation, ensuring that systems meet the increasing demands of performance, safety, and sustainability.
1.3) HOW DOES CONTROL THEORY IN ENGINEERING AND TECHNOLOGY HELP ADDRESS REAL-WORLD PROBLEMS?
Control theory in engineering and technology provides a framework for designing systems that regulate behavior to achieve desired outcomes, even in the presence of disturbances and uncertainties. This theory has been fundamental in addressing numerous real-world problems by ensuring systems are stable, efficient, and reliable across various industries.
In the energy sector, control theory has played a key role in integrating renewable energy sources into existing power grids, which is critical in combating climate change. Systems such as wind turbines and solar panels use control algorithms to optimize power generation and stabilize energy outputs. For example, maximum power point tracking (MPPT) techniques ensure that solar panels operate at peak efficiency despite variations in sunlight, while pitch control systems in wind turbines adjust blade angles to maximize energy capture in varying wind conditions (Zhu et al., 2013). These applications have significantly enhanced the sustainability and efficiency of energy systems worldwide.
In the transportation industry, control theory underpins the design of safety and efficiency features in vehicles, including anti-lock braking systems (ABS), cruise control, and traction control. These systems use feedback mechanisms to adjust vehicle performance in real-time, ensuring safety and stability under dynamic conditions. In aviation, control theory enables the design of autopilot systems that maintain stable flight paths, reduce pilot workload, and improve flight safety by dynamically adjusting to factors such as wind turbulence and atmospheric changes (Stevens & Lewis, 2003). More recently, control theory has been crucial in the development of autonomous vehicles, where it is applied in path planning, obstacle avoidance, and speed regulation, allowing vehicles to navigate complex environments with minimal human intervention.
In industrial automation, control theory addresses the need for precise and efficient manufacturing processes. Process control systems regulate variables such as temperature, pressure, and flow in industries ranging from chemical processing to semiconductor manufacturing. Techniques like Model Predictive Control (MPC) allow for real-time optimization of production systems, enabling industries to achieve higher yields, lower energy consumption, and improved product quality (Seborg et al., 2010). This has made industries more competitive and sustainable by reducing waste and operating costs.
Healthcare is another field where control theory has had transformative impacts. It is central to the development of advanced medical devices such as insulin pumps, artificial hearts, and ventilators. These devices rely on adaptive control algorithms to adjust their operation in real time, ensuring they meet patient-specific needs. For instance, insulin pumps use feedback loops to regulate blood glucose levels by monitoring sugar levels and administering precise insulin doses. Similarly, modern ventilators adjust airflow and pressure based on the patient’s respiratory requirements, improving survival rates in critical care (Astrom & Murray, 2012).
In robotics and automation, control theory enables robots to perform complex tasks with precision and adaptability. Robots used in manufacturing, surgery, and hazardous environments rely on control systems to ensure accurate positioning, motion control, and task execution. Surgical robots, for example, use control algorithms to enhance the dexterity and precision of human surgeons, reducing risks associated with invasive procedures (Ogata, 2010). This has expanded the scope of robotics into fields requiring high accuracy and reliability.
Urban infrastructure has also benefited from control theory through advancements in smart traffic management systems. Adaptive traffic control systems use feedback from sensors to optimize traffic flow, reduce congestion, and lower fuel consumption. By dynamically adjusting traffic signals based on real-time data, these systems have improved urban mobility and reduced environmental impacts in cities facing growing population pressures.
Communication systems apply control theory to maintain signal stability and quality in networks prone to noise and interference. Feedback and feedforward control mechanisms are used to regulate transmission power and reduce signal degradation. These technologies have been essential in ensuring the reliability of modern communication systems, including mobile networks, satellite communications, and internet data transfer (Proakis & Manolakis, 1996).
The control theory has therefore addressed real-world problems by providing tools to design systems that operate reliably, adapt to changing conditions, and achieve optimal performance. From energy sustainability and transportation safety to industrial automation, healthcare, robotics, and communication systems, its applications have improved efficiency, enhanced safety, and driven innovation in critical areas of society.
Control theory continues to evolve, offering even more sophisticated solutions to complex real-world problems in various domains of engineering and technology. Below is a more detailed exploration of its applications:
In modern energy systems, control theory addresses grid stability and the efficient management of distributed energy resources. Smart grids employ advanced control systems to balance electricity supply and demand in real time. For instance, demand-side management uses predictive control algorithms to optimize energy consumption patterns during peak hours, minimizing energy waste and reducing operational costs. In addition, battery energy storage systems use state-of-charge control strategies to store excess energy during off-peak periods and release it when demand is high. These systems enable a more resilient and reliable energy infrastructure (Luo et al., 2015).
In climate control and HVAC systems, control theory enhances building energy efficiency and occupant comfort. By using feedback mechanisms to adjust heating, ventilation, and air conditioning (HVAC) systems based on sensor inputs, control algorithms can regulate indoor environments more effectively. Predictive control strategies can also consider external factors like weather forecasts to reduce energy consumption while maintaining optimal comfort levels. This has become a vital tool in achieving green building certifications and reducing carbon footprints (Wang & Ma, 2008).
In the aerospace industry, control theory addresses challenges related to spacecraft navigation, stability, and performance in hostile environments. Attitude control systems, for instance, ensure that satellites and space probes maintain their orientation in orbit using precise feedback from gyroscopes and accelerometers. Advanced control algorithms like robust and adaptive control handle uncertainties in the system, such as variable gravitational forces or mechanical disturbances. This has been critical for missions like the Mars rovers, where maintaining operational stability is crucial for data collection and transmission (Beard & McLain, 2012).
The biomedical field applies control theory in advanced prosthetics and rehabilitation devices. Prosthetic limbs equipped with control algorithms can interpret neural signals from users and adapt their movements in real-time. Similarly, exoskeletons for rehabilitation use control systems to assist patients with walking, ensuring stability and replicating natural gait patterns. These innovations are helping individuals with disabilities regain mobility and improve their quality of life (Ferris et al., 2005).
In chemical engineering, control theory is used to optimize reaction processes in real-time. Advanced control systems regulate critical parameters such as temperature, pressure, and chemical concentrations in reactors, ensuring that production yields meet quality standards. In pharmaceutical manufacturing, control theory ensures the consistency of drug formulations through precise regulation of mixing and blending processes. This is especially important for industries where safety and compliance with regulatory standards are paramount (Seborg et al., 2010).
The application of control theory in environmental engineering has contributed to sustainable water and waste management. Wastewater treatment plants, for example, use control systems to regulate biological and chemical processes for treating water. Adaptive control strategies adjust aeration levels and chemical dosing based on fluctuations in water quality, ensuring compliance with environmental standards. These systems help protect ecosystems while reducing operational costs (Olsson & Newell, 1999).
In autonomous systems and robotics, control theory has advanced the development of intelligent systems that interact with dynamic environments. For instance, drones use control algorithms to maintain stability during flight while adapting to changing wind conditions. Control systems in industrial robots enable precise motion control for tasks like assembly and welding. More complex robots, such as humanoids, use control strategies to achieve balance, navigate uneven terrains, and perform human-like tasks. This has paved the way for robotics to become integral in fields like disaster response, exploration, and eldercare (Siciliano & Khatib, 2016).
Control theory also addresses challenges in financial engineering, where it is applied in algorithmic trading and risk management. Portfolio optimization algorithms use predictive control strategies to adjust investment allocations dynamically, maximizing returns while minimizing risk under uncertain market conditions. These applications demonstrate the versatility of control theory beyond physical systems, extending its utility to economic and social systems as well (Boyd & Vandenberghe, 2004).
1.4) WITH PRACTICAL EXAMPLES, HOW HAS CONTROL THEORY IN ENGINEERING AND TECHNOLOGY HELPED ADDRESS REAL-WORLD PROBLEMS?
Control theory has been instrumental in solving real-world problems across multiple engineering and technology domains, offering practical solutions to challenges involving dynamic systems. It provides a structured way to design systems that behave predictably under varying conditions, thereby improving efficiency, safety, and reliability.
A notable example of control theory application is in power grid stabilization and renewable energy integration. Renewable energy sources, such as solar panels and wind turbines, are inherently variable due to fluctuations in sunlight and wind. To address this, advanced control algorithms such as Maximum PowerPoint Tracking (MPPT) have been developed. These algorithms dynamically adjust the operating point of solar panels to maximize energy output regardless of changing environmental conditions (Zhu et al., 2013). Similarly, wind turbines use pitch control systems, which adjust the angle of the blades to optimize energy capture and prevent damage during high winds. These control strategies have made it feasible to incorporate renewable energy into power grids, reducing dependence on fossil fuels and addressing global energy sustainability challenges.
In the automotive industry, control theory has significantly enhanced vehicle safety and performance. One practical application is the Anti-lock Braking System (ABS), which prevents wheel lockup during braking by continuously monitoring wheel speed and adjusting brake pressure accordingly. This feedback control mechanism ensures that the vehicle maintains traction and can be steered during emergency braking, reducing accident rates (Stevens & Lewis, 2003). Another example is the cruise control system, which maintains a vehicle’s speed by automatically adjusting the throttle position based on real-time feedback from speed sensors. These innovations have made driving safer and more convenient.
The aviation industry has also benefited extensively from control theory, particularly in autopilot systems. Autopilots use control algorithms to maintain a stable flight path, regulate altitude, and ensure proper navigation, even in the presence of turbulence or other disturbances. For example, the proportional-integral-derivative (PID) controller is widely used to adjust the aircraft’s pitch, roll, and yaw angles to achieve stable flight conditions. This reduces pilot workload and enhances passenger safety. Additionally, control theory is critical in the design of fly-by-wire systems, which replace traditional mechanical controls with electronic ones, allowing aircraft to respond more quickly and accurately to pilot inputs (Stevens & Lewis, 2003).
In healthcare, control theory has been transformative in medical device development. Insulin pumps for diabetes management use control algorithms to monitor blood glucose levels and administer precise doses of insulin, ensuring that patients maintain stable glucose levels. Similarly, ventilators, which became particularly critical during the COVID-19 pandemic, rely on control systems to regulate airflow, pressure, and oxygen concentration based on a patient’s respiratory needs. These devices have saved countless lives by providing real-time adjustments to meet patient-specific conditions (Astrom & Murray, 2012).
In the realm of industrial automation, control theory has revolutionized manufacturing processes. For example, chemical plants use process control systems to regulate reaction conditions such as temperature, pressure, and flow rates, ensuring consistent product quality. A specific case is in oil refineries, where distillation columns are controlled using feedback systems to maintain optimal separation of crude oil into various products. These systems minimize energy consumption and operational costs while improving efficiency (Seborg et al., 2010).
Control theory has also addressed problems in urban traffic management. Adaptive traffic signal control systems use real-time data from sensors to optimize traffic flow at intersections. For instance, the Split Cycle Offset Optimization Technique (SCOOT) adjusts signal timings dynamically based on traffic conditions, reducing congestion and travel time. This has been implemented in cities worldwide, such as London and Singapore, where it has improved urban mobility and reduced environmental impact by lowering fuel consumption and emissions (Wang & Ma, 2008).
In robotics, control theory enables robots to perform tasks with precision and adaptability. Robotic arms used in manufacturing rely on control systems to achieve accurate positioning and motion. For instance, assembly robots in the automotive industry use feedback control to adjust their movements in real-time, ensuring high precision during welding or part placement. Additionally, in the medical field, surgical robots like the da Vinci system use control algorithms to translate a surgeon’s hand movements into precise actions, enhancing surgical accuracy and reducing patient recovery times (Ogata, 2010).
Aerospace applications also illustrate the power of control theory, particularly in spacecraft navigation and stability. Satellites use attitude control systems to maintain their orientation in orbit, enabling accurate positioning for communication, imaging, or scientific observation. These systems use sensors such as gyroscopes and accelerometers to monitor satellite motion and adjust thrusters or reaction wheels accordingly. This application was critical in the Mars rover missions, where robust control systems ensured the rovers could navigate and operate autonomously in harsh and unpredictable environments (Beard & McLain, 2012).
Control theory has played a significant role in improving the efficiency and reliability of communication systems, particularly in modern wireless networks. For example, control algorithms are used in adaptive modulation and coding schemes to optimize data transmission based on channel conditions. When a mobile device experiences poor signal quality due to interference or distance from the base station, the system dynamically adjusts the data rate and power levels to maintain a stable connection. This application has been critical in the evolution of 5G networks, enabling faster and more reliable communication even in densely populated urban areas (Tse & Viswanath, 2005).
In rail transportation, control theory is applied in train control systems, such as Automatic Train Control (ATC) and Positive Train Control (PTC). These systems monitor train positions and speeds in real-time, ensuring safe operation by preventing collisions and derailments. For instance, PTC systems use sensors and feedback loops to automatically slow down or stop trains when they approach dangerous sections of track. This technology has enhanced safety and operational efficiency in railway networks worldwide, especially in high-speed rail systems (Ding et al., 2014).
In supply chain and logistics, control theory has been applied to optimize inventory management and delivery systems. For example, companies like Amazon use predictive control algorithms in their warehouse operations to manage inventory levels efficiently and fulfill customer orders promptly. These systems monitor stock levels in real time and adjust procurement schedules dynamically, reducing overstock and understock issues. Additionally, route optimization for delivery vehicles is achieved through control strategies that consider traffic conditions, fuel efficiency, and delivery time constraints, significantly reducing operational costs (Disney & Towill, 2003).
In marine engineering, control theory is used to stabilize ships and offshore platforms. Dynamic positioning systems (DPS) rely on control algorithms to keep vessels stationary or on a precise path despite external disturbances like wind and currents. This is particularly important in offshore drilling operations, where platforms must maintain stability for safety and accuracy. These systems use real-time feedback from sensors to adjust thrusters and maintain the desired position, enabling operations in deep waters and challenging environments (Fossen, 2011).
Control theory also addresses challenges in manufacturing automation through collaborative robotics, commonly referred to as cobots. Unlike traditional industrial robots, cobots work alongside human operators to perform tasks that require precision and flexibility. Control algorithms ensure that the cobots adapt to the actions of human workers, maintaining safety and efficiency. For instance, in automotive assembly lines, cobots assist workers in lifting heavy components and welding joints with sub-millimeter accuracy. This collaboration reduces the physical strain on workers and improves overall productivity (Kemp et al., 2007).
In environmental monitoring, control theory has been applied to develop sensor networks for tracking pollution levels. For example, in air quality monitoring systems, control algorithms process data from multiple sensors to provide real-time feedback on pollution levels across urban areas. These systems can also activate measures such as increasing ventilation in enclosed spaces or adjusting traffic patterns to reduce emissions during peak pollution hours. Such applications are crucial for addressing environmental challenges and improving public health in cities (Corke et al., 2010).
In agriculture, control theory supports precision farming practices, where sensors and actuators regulate irrigation, fertilization, and pest control. For example, smart irrigation systems use feedback from soil moisture sensors to determine the exact amount of water needed for crops, reducing water wastage and improving yield. Drones equipped with control algorithms can also monitor crop health by analyzing aerial images and spraying fertilizers or pesticides in specific areas. These applications have transformed traditional farming into a more efficient and sustainable practice (Bates & Granger, 2020).
Control theory has also been pivotal in intelligent building systems, where it helps manage energy usage and occupant comfort. For instance, building management systems (BMS) use control algorithms to integrate heating, ventilation, air conditioning, lighting, and security systems. These systems optimize energy usage based on real-time occupancy data and external environmental conditions. In large commercial buildings, BMS can reduce energy consumption by up to 30% while maintaining a comfortable indoor environment (Wang & Ma, 2008).
Finally, in the field of smart cities, control theory underpins traffic management systems. Adaptive traffic signal control systems use real-time data from cameras and sensors to adjust traffic light timings dynamically. This reduces congestion and minimizes travel times, particularly during peak hours. For example, cities like Singapore and Los Angeles have implemented such systems to manage complex urban traffic networks, significantly reducing fuel consumption and greenhouse gas emissions (Zhang et al., 2019).
1.5) SIGNIFICANCE OF THE CONTROL THEORY IN THE 21ST CENTURY, PARTICULARLY IN THE FIELDS OF ENGINEERING AND TECHNOLOGY
Control theory has become an indispensable framework in the 21st century, significantly advancing engineering and technology. Its impact is evident in the development of systems and technologies that underpin modern society, contributing to increased efficiency, reliability, and sustainability in numerous applications.
In the field of engineering, control theory has been a cornerstone of automation and robotics, which have revolutionized industrial processes. Automated manufacturing systems rely on control theory to ensure precise and efficient operation. For example, advanced controllers like Proportional-Integral-Derivative (PID) controllers are used in assembly lines to regulate the speed, position, and torque of robotic arms. These systems ensure that repetitive tasks are executed with high accuracy, improving productivity and reducing waste (Ogata, 2010). Furthermore, adaptive control techniques allow robots to adjust to changing conditions in real time, enabling their use in dynamic and unstructured environments, such as construction sites and disaster recovery operations (Siciliano & Khatib, 2016).
In technology, control theory has played a pivotal role in the development of modern communication systems. Wireless networks, including 5G, employ control algorithms to optimize data transmission, allocate resources, and manage interference. For instance, power control algorithms are used to adjust the transmission power of devices, ensuring minimal interference and efficient use of the spectrum. This has enabled faster, more reliable communication systems that support applications such as the Internet of Things (IoT) and augmented reality (Tse & Viswanath, 2005). These advancements are critical in a highly connected world where seamless communication is integral to daily life.
Control theory has also been central to advancements in transportation systems. Autonomous vehicles rely heavily on control systems to navigate safely and efficiently. These systems integrate feedback loops to process sensor data and make real-time decisions about speed, direction, and obstacle avoidance. By enhancing safety and reducing human error, control theory has brought autonomous vehicles closer to becoming a reality in mainstream transportation (Beard & McLain, 2012). Additionally, control algorithms are employed in traffic management systems to optimize traffic flow, reduce congestion, and minimize environmental impact. For example, adaptive traffic signal control systems use control principles to adjust signal timings based on real-time traffic conditions, significantly improving urban mobility (Zhang et al., 2019).
In the energy sector, control theory has enabled the efficient operation of renewable energy systems. Wind turbines, for instance, use control algorithms to adjust the pitch of their blades in response to changes in wind speed, ensuring optimal energy production while protecting the system from damage during high winds. Similarly, solar power systems employ Maximum Power Point Tracking (MPPT) controllers to maximize energy conversion efficiency under varying sunlight conditions (Zhu et al., 2013). These applications have been critical in addressing the global demand for sustainable and reliable energy solutions.
Healthcare has also benefited immensely from control theory. Advanced medical devices, such as insulin pumps and ventilators, use feedback control systems to maintain precise levels of insulin delivery or airflow, ensuring patient safety and improving health outcomes. Furthermore, control theory underpins the operation of robotic surgical systems, which offer unparalleled precision in minimally invasive procedures, reducing recovery times and enhancing patient care (Astrom & Murray, 2012).
Control theory’s contribution to environmental sustainability is another area of significance. Water treatment plants use control systems to optimize chemical dosing and aeration processes, ensuring compliance with environmental regulations while minimizing operational costs. Additionally, smart grid technologies rely on control theory to balance electricity supply and demand, integrate renewable energy sources, and improve the overall efficiency of power distribution networks (Seborg et al., 2010). These systems are vital in addressing the challenges of climate change and resource scarcity.
In aerospace engineering, control theory is fundamental to the stability and performance of aircraft and spacecraft. Autopilot systems use control algorithms to maintain altitude, speed, and heading, reducing pilot workload and enhancing safety. In space exploration, control theory governs the navigation and attitude control of spacecraft, enabling precise maneuvers for missions such as landing on Mars or docking with the International Space Station (Ogata, 2010).
Moreover, control theory has driven the evolution of smart technologies and IoT devices, which are increasingly integrated into everyday life. Smart thermostats, for example, use feedback loops to adjust heating and cooling systems based on occupancy and external weather conditions, improving energy efficiency. Similarly, wearable health monitors use control systems to provide real-time feedback on vital signs, empowering users to make informed decisions about their health (Wang & Ma, 2008).
The significance of control theory in the 21st century is profound, particularly in engineering and technology. By enabling precision, efficiency, and adaptability, it has advanced industries such as automation, energy, healthcare, transportation, and telecommunications. Its applications address modern challenges, including climate change, urbanization, and the demand for sustainable and efficient systems, making it a cornerstone of progress in an increasingly complex world.
Control theory remains at the core of solving contemporary challenges in engineering and technology, enabling groundbreaking advancements across diverse industries. Beyond automation, energy management, healthcare, and transportation, control theory has expanded its influence into areas such as artificial intelligence (AI), environmental protection, cybersecurity, and space exploration.
In artificial intelligence, control theory is pivotal in designing systems that exhibit intelligent behavior. Reinforcement learning, a machine learning paradigm, is deeply influenced by optimal control principles. For instance, self-driving cars leverage control theory to train models that balance safety and efficiency during navigation. By combining control theory with neural networks, AI systems are designed to predict and adapt to dynamic environments, offering real-time solutions for challenges in industries ranging from supply chain management to personalized healthcare (Sutton & Barto, 2018).
Environmental protection has benefited from the application of control theory to monitor and manage complex ecological systems. Advanced control strategies are used in precision agriculture to optimize the use of water, fertilizers, and pesticides. For example, irrigation systems with embedded control algorithms determine the optimal amount of water required for crops, reducing waste and conserving resources (Jones, 2004). Similarly, control systems in wastewater treatment plants regulate the flow of pollutants, ensuring cleaner water outputs. These systems contribute to sustainability by minimizing environmental harm while maintaining high productivity.
In the realm of cybersecurity, control theory offers innovative approaches to detect and mitigate threats in real-time. Cyber-physical systems, such as smart grids and industrial control systems, use control-theoretic models to detect anomalies and implement automated responses to attacks. For example, distributed control strategies are employed to isolate infected nodes in a network, ensuring system integrity while maintaining functionality (Zhu & Basar, 2015). This approach has become increasingly critical as cyber threats grow more sophisticated, impacting sectors such as finance, energy, and defense.
In space exploration, the role of control theory continues to grow, enabling the development of advanced spacecraft and planetary exploration missions. Modern space missions rely on robust control algorithms for trajectory planning, satellite stabilization, and docking procedures. For example, NASA’s Mars rovers, such as Perseverance, utilize control algorithms to navigate challenging terrain autonomously. These systems integrate data from sensors and cameras to make real-time adjustments, ensuring mission success even in the absence of direct human intervention (Bekey, 2005).
The rise of smart cities also highlights the importance of control theory. Urban environments are becoming increasingly complex, requiring coordinated systems to manage energy, transportation, and public services. Control theory underpins the operation of smart grids, which dynamically balance electricity supply and demand across urban areas. It also facilitates intelligent building management systems, where sensors and actuators control heating, cooling, lighting, and security based on occupancy and weather conditions. This not only improves energy efficiency but also enhances urban living standards (Ghaffarianhoseini et al., 2016).
Furthermore, control theory has enabled transformative advancements in quantum computing. Quantum error correction—a fundamental aspect of building reliable quantum computers—is grounded in control theory. The stabilization of quantum states requires feedback control to counteract noise and decoherence, making quantum systems more robust and scalable (Doherty et al., 2000). This breakthrough has the potential to revolutionize fields such as cryptography, drug discovery, and materials science.
In renewable energy, control theory has been extended to include predictive and adaptive techniques. Wind farms use predictive control to adjust turbine operations based on wind forecasts, maximizing energy output while minimizing wear and tear. Similarly, solar energy systems utilize adaptive control to optimize performance under fluctuating weather conditions. Energy storage systems, such as batteries and pumped hydro, are also managed using control algorithms to ensure efficient energy dispatch and grid stability during peak demand (Blaabjerg et al., 2021).
The field of biomedicine has seen a remarkable application of control theory in prosthetics and assistive devices. Modern prosthetic limbs employ control algorithms to interpret neural signals, enabling intuitive and precise movements for users. Closed-loop control systems are also used in neuroprosthetics to restore motor functions in patients with spinal cord injuries, demonstrating the profound impact of control theory on improving quality of life (Popovic et al., 2014).
Thus, the 21st century has seen an unprecedented expansion of control theory into diverse and transformative applications. By integrating with cutting-edge technologies, such as AI, quantum computing, and IoT, control theory addresses real-world problems with precision and adaptability. Its role in advancing sustainability, cybersecurity, healthcare, and space exploration underscores its relevance in tackling global challenges, making it an indispensable tool for innovation and problem-solving in engineering and technology.
1.6) SCHOLAR’S CRITICISM OF THE CONTROL THEORY UNDER ENGINEERING AND TECHNOLOGY
The Control Theory, which is pivotal in the fields of engineering and technology, has received significant criticism from scholars who have examined its theoretical underpinnings and practical applications.
One major critique is the assumption of linearity in many control systems, which fails to account for the complexities and nonlinear dynamics of real-world systems. According to Slotine and Li (1991), while linear control theory provides a foundational framework, its application to nonlinear systems often leads to oversimplification, which can result in suboptimal or even unstable system behavior. This limitation has driven the development of nonlinear control theories, yet the practical implementation of these theories often remains challenging due to their mathematical complexity.
Another criticism is the reliance on idealized models in control system design. Zames (1981) highlights that the robustness of control systems is compromised when discrepancies exist between the theoretical model and actual system behavior. This issue is particularly pronounced in adaptive control systems, where environmental uncertainties and parameter variations can render the control strategies ineffective.
Additionally, traditional control theory is often criticized for its lack of integration with modern computational methods. Despite advances in artificial intelligence and machine learning, which can significantly enhance system adaptability and decision-making, these techniques are not fully incorporated into classical control frameworks. Lewis et al. (2012) argue that the future of control theory lies in integrating intelligent systems, yet this integration remains limited in practice due to a lack of interdisciplinary approaches.
Moreover, the applicability of control theory to large-scale, interconnected systems has been questioned. Systems such as smart grids and autonomous networks present challenges in scalability and coordination, as highlighted by Khalil and Grizzle (2002). The centralized approach inherent in traditional control theory often fails to address the decentralized and distributed nature of modern technological systems, leading to inefficiencies and vulnerabilities.
In summary, while control theory has provided a robust foundation for engineering and technology, scholars have critiqued its limitations in addressing nonlinearities, modeling inaccuracies, lack of computational integration, and challenges in managing large-scale systems. These criticisms emphasize the need for continued evolution and interdisciplinary collaboration to address the demands of modern technological advancements. The following are specific criticisms.
1.6.1) Limited Consideration of Human Factors in System Design
Control theory often neglects the human element in system design and operation, especially in human-machine interactions. Sheridan (1992) critiques traditional control frameworks for failing to address the cognitive and behavioral aspects of human operators. In scenarios such as aviation, healthcare robotics, and autonomous vehicles, the omission of human factors has led to mismatches between the system’s behavior and the operator’s expectations, sometimes causing safety risks.
1.6.2) Overemphasis on Stability at the Expense of Performance
While stability is a cornerstone of control theory, many scholars argue that this focus can overshadow the importance of system performance in dynamic and rapidly changing environments. Doyle et al. (1992) point out that optimal control strategies must balance robustness and performance, yet many traditional approaches prioritize stability excessively, leaving the system less responsive to unexpected disturbances.
1.6.3) Inflexibility in Handling Unstructured Environments
Control systems are often designed for structured environments where variables and disturbances are relatively predictable. However, in real-world applications like robotics, autonomous navigation, and industrial automation, unstructured environments are common. Åström and Murray (2008) criticize classical control methods for their lack of adaptability, suggesting that they are ill-suited for situations where the system must operate under uncertain or rapidly changing conditions.
1.6.4) Insufficient Integration with Emerging Technologies
The rapid development of technologies such as the Internet of Things (IoT), cloud computing, and quantum computing poses challenges for traditional control theory. For example, IoT environments involve vast numbers of interconnected devices generating large amounts of data in real-time. Wan et al. (2015) argue that classical control theory lacks the scalability and computational power to handle such large-scale, data-intensive systems, necessitating new frameworks like data-driven or decentralized control.
1.6.5) Ethical and Security Challenges
Control systems increasingly influence critical sectors like energy, healthcare, and defense, raising ethical and security concerns. For example, as control systems become more automated and reliant on artificial intelligence, the potential for algorithmic biases or malicious attacks increases. Anderson et al. (2017) point out that classical control theory does not inherently account for these risks, making it necessary to integrate ethical considerations and cybersecurity measures into control system design.
1.6.6) Inadequate Handling of Multiscale and Multiphysics Systems
Modern engineering challenges often involve systems that operate across multiple spatial and temporal scales, such as climate models, biomedical devices, and nanotechnology. Traditional control theory, as noted by Meurer et al. (2012), struggles to address the complexity of multiphysics systems where interactions across different domains (e.g., mechanical, electrical, and thermal) must be considered simultaneously.
1.6.7) Reliance on Fixed Parameters and Rigid Designs
Traditional control theory relies heavily on fixed parameters and predefined models, which can limit its applicability in systems that require flexibility or self-optimization. Advances in adaptive and self-tuning control have addressed some of these issues, but as noted by Ioannou and Sun (2012), these methods remain underutilized in many practical applications due to their computational intensity and complexity.
1.6.8) Challenges in Hybrid Systems
Hybrid systems that combine discrete and continuous dynamics, such as automated manufacturing lines and cyber-physical systems, present unique challenges for control theory. Lunze and Lamnabhi-Lagarrigue (2009) highlight the difficulty of integrating discrete event systems with traditional continuous control frameworks, resulting in gaps in design and performance.
1.7) THE ACADEMIC TIMES JOURNAL AIMS TO ADVANCE THE UNDERSTANDING AND APPLICATION OF CONTROL THEORY FOR POLICY-MAKING THROUGH STAKEHOLDER ENGAGEMENT, OFFERING CONSTRUCTIVE SCHOLARLY CRITIQUES, AND LEVERAGING CRITICISMS TO FACILITATE EFFECTIVE DECISION-MAKING.
The Academic Times Journal aims to advance the understanding and application of control theory in policy-making by employing a multifaceted approach that includes stakeholder engagement, the provision of constructive scholarly critiques, and the strategic leveraging of criticisms to facilitate effective decision-making.
Stakeholder engagement forms a cornerstone of the journal’s strategy. By actively involving policymakers, academics, and practitioners, the journal ensures a collaborative process that bridges theoretical concepts and practical policy applications. This engagement fosters the adaptation of control theory to address real-world challenges, making it a vital tool for enhancing the efficiency, adaptability, and effectiveness of policy decisions (Sterman, 2000). Through this participatory approach, stakeholders gain insights into how control theory can be applied to complex systems, such as governance, public health, and environmental sustainability.
The journal also emphasizes the importance of offering constructive scholarly critiques. By facilitating rigorous peer-reviewed analysis, The Academic Times Journal encourages contributors to critically examine the assumptions, methodologies, and applications of control theory. These critiques contribute to refining the theoretical framework and extending its relevance to contemporary policy challenges (Forrester, 1961). Such scholarly discourse strengthens the validity and applicability of control theory, ensuring it remains a robust analytical tool for decision-makers.
Finally, the journal leverages criticisms as a mechanism to promote effective decision-making. By applying the feedback loop principles inherent in control theory, criticisms are analyzed and transformed into actionable insights. This iterative process drives the continuous refinement of theoretical models and their application, enabling the journal to address dynamic policy issues such as economic crises, climate change, and technological innovation (Wiener, 1948). Criticism, in this context, is not viewed as a hindrance but as a critical driver for improvement and innovation in policy-making frameworks.
Control theory has been a fundamental framework in engineering and technology, guiding the design, analysis, and operation of dynamic systems. Its principles enable systems to maintain stability, optimize performance, and adapt to external changes. In engineering, control theory finds applications in automation, robotics, telecommunications, and process control, where feedback mechanisms ensure precision and efficiency. Similarly, in technology, control systems play a pivotal role in artificial intelligence, machine learning, and IoT devices, offering dynamic adaptability and responsiveness to real-time data. These applications demonstrate the transformative power of control theory in enhancing technological innovation and efficiency.
The Academic Times Journal recognizes the potential of control theory beyond its traditional domains and aims to advance its application in policy-making. By focusing on stakeholder engagement, the journal ensures a collaborative environment where policymakers, academics, and practitioners can work together to align theoretical concepts with real-world applications. This approach bridges the gap between theory and practice, fostering the adaptation of control systems to address complex policy challenges such as climate change, economic stability, and public health management.
Through constructive scholarly critiques, The Academic Times Journal encourages rigorous evaluation of control theory frameworks, promoting innovation and relevance. By inviting critical analysis, the journal refines the theoretical underpinnings of control theory, ensuring its continued applicability to contemporary issues. Additionally, leveraging criticisms as a learning tool aligns with the iterative principles of control theory itself, transforming feedback into actionable insights that drive effective decision-making. This process enhances the robustness of policies and their ability to adapt to changing circumstances.
The control theory remains a cornerstone of engineering and technology, driving advancements in automation, optimization, and adaptability. The Academic Times Journal extends these principles to the realm of policy-making, emphasizing collaboration, scholarly critique, and iterative learning. By doing so, it facilitates the integration of control theory into governance, enabling policymakers to design responsive and effective strategies. This interdisciplinary approach not only enriches the theoretical landscape of control theory but also ensures its practical impact on addressing global challenges and improving decision-making processes.
Control theory, a foundational concept in engineering and technology, has revolutionized how dynamic systems are designed, monitored, and managed. By providing feedback mechanisms and control structures, it enables systems to achieve stability, optimize performance, and adapt to changes in their environment. In engineering, its applications span across diverse fields such as robotics, process control, telecommunications, and automotive systems, ensuring precision and reliability in operations. Similarly, in technology, control theory underpins advancements in artificial intelligence, machine learning, and the Internet of Things, enabling systems to respond dynamically to real-time data and shifting conditions. These contributions demonstrate its indispensable role in modern innovation and its potential to address complex challenges.
The Academic Times Journal expands the relevance of control theory beyond its technical origins by exploring its applications in policy-making. Recognizing the interdisciplinary nature of policy challenges, the journal focuses on fostering stakeholder engagement as a critical tool for integrating control theory into governance frameworks. Through collaborative initiatives, it bridges the gap between theorists, policymakers, and practitioners, ensuring that the insights from control theory are effectively translated into actionable strategies. This engagement provides an opportunity to adapt control systems to address multifaceted global issues, such as climate change, economic inequalities, and public health crises.
In addition to promoting collaboration, the journal emphasizes the importance of constructive scholarly critiques. These critiques allow for a rigorous evaluation of control theory applications, exposing limitations and uncovering opportunities for innovation. By encouraging a continuous process of critical examination, the journal ensures that control theory remains relevant and adaptable to emerging challenges. Critical analysis often leads to new advancements, such as integrating behavioral insights or leveraging data-driven technologies like artificial intelligence to refine control systems for real-world scenarios.
The journal also champions the use of criticisms as a catalyst for learning and development. This approach aligns with the iterative nature of control theory itself, where feedback loops are used to adjust and improve system performance. Leveraging criticisms in this way allows for the development of policies that are resilient, adaptive, and informed by practical insights. For example, using simulations and system dynamics models, policymakers can test and refine their strategies before implementation, ensuring that real-world outcomes align with intended goals.
Moreover, The Academic Times Journal situates control theory within the context of pressing global challenges. It explores how its principles can be used to design adaptive policies for issues like climate governance, where control systems can monitor emissions and dynamically adjust strategies to achieve environmental goals. Similarly, in public health, control theory provides a framework for managing crises such as pandemics, optimizing resource allocation, and ensuring the timely implementation of interventions. These examples highlight the transformative potential of control theory in addressing global policy issues.
The Academic Times Journal also prioritizes educational outreach to broaden the accessibility of control theory. By producing accessible publications, developing training programs, and partnering with academic institutions, it ensures that the concepts of control theory are disseminated widely among policymakers, researchers, and practitioners. This effort not only demystifies complex systems but also equips decision-makers with the tools needed to apply control theory effectively in their domains.
In summary, control theory remains a cornerstone of engineering and technology, providing essential frameworks for stability, optimization, and adaptability. The Academic Times Journal builds upon these principles, extending their application to policy-making by emphasizing stakeholder collaboration, scholarly critique, and iterative learning. By doing so, it bridges the gap between theory and practice, ensuring that control theory contributes meaningfully to addressing contemporary global challenges and enhancing decision-making processes across multiple sectors. Through its innovative approach, the journal reinforces the practical relevance of control theory and its ability to drive transformative change in governance and policy implementation.