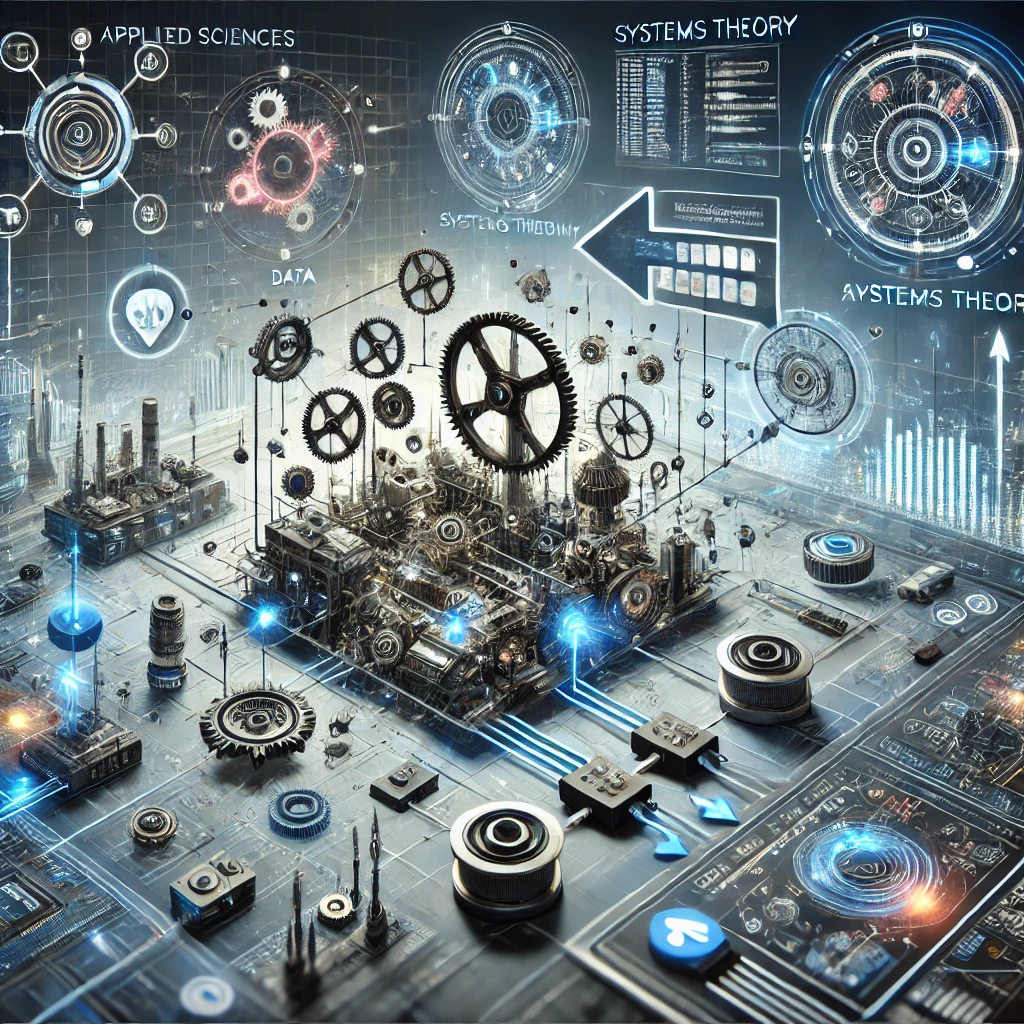
1.1) OVERVIEW AND ORIGIN OF SYSTEM THEORY IN ENGINEERING AND TECHNOLOGY
Systems theory in engineering and technology is a framework that emphasizes the interconnectedness and interdependence of components within a system. It originated from the need to address the limitations of reductionist approaches, which isolate individual components without considering the broader context of their interactions within the system. The theory provides a holistic view of systems, considering how their parts work together to produce outcomes that cannot be understood merely by analyzing the parts independently. It plays a crucial role in engineering, especially in fields like control systems, automation, robotics, and network design, where understanding the dynamic interactions of components is essential for optimizing system performance.
The origin of systems theory can be traced back to the contributions of several key scholars, most notably Ludwig von Bertalanffy, Norbert Wiener, and Ross Ashby. These figures laid the foundation for systems theory by developing ideas that reshaped how engineers and scientists understand and design complex systems.
Ludwig von Bertalanffy is widely regarded as one of the foremost contributors to the development of systems theory. In the 1940s, Bertalanffy introduced General Systems Theory (GST) as a way to study systems across different disciplines in a unified manner. His work challenged the traditional reductionist approach, which focused on breaking systems down into individual components for analysis. Instead, Bertalanffy proposed that systems must be viewed as wholes, where the interactions between components are just as important as the components themselves. Bertalanffy emphasized that systems exhibit emergent properties—characteristics or behaviors that arise from the interactions of components, which cannot be understood by studying the parts in isolation. His ideas provided a foundation for the application of systems theory in engineering and other fields where the focus is on complex, dynamic systems (Bertalanffy, 1968).
Norbert Wiener, a mathematician and engineer, further developed systems theory by introducing the concept of cybernetics in the 1940s. Cybernetics focuses on the study of communication and control in systems, whether mechanical, biological, or social. Wiener’s work on feedback mechanisms and the regulation of systems was instrumental in shaping the way engineers approach system design. His research showed how systems, whether in engineering or biology, use feedback loops to maintain stability or adapt to changes. Feedback, a central concept in cybernetics, refers to the process by which a system receives information about its performance and adjusts its behavior to achieve desired outcomes. This idea has had a significant impact on control systems engineering, where feedback is used to regulate processes and ensure that systems maintain desired performance despite changes in their environment (Wiener, 1961).
Ross Ashby’s contributions to systems theory further deepened the understanding of self-regulation and adaptation in systems. In his book An Introduction to Cybernetics (1956), Ashby explored the concept of homeostasis, which refers to the ability of a system to maintain internal stability despite changes in external conditions. His work demonstrated that systems could be designed to automatically adjust their behavior in response to external disturbances, ensuring their continued functionality. Ashby’s ideas are particularly relevant in fields like control engineering, robotics, and automation, where systems need to respond dynamically to varying inputs. He showed how self-regulation and feedback mechanisms could be integrated into systems to maintain stability and adapt to changes, paving the way for the development of adaptive control systems and intelligent machines (Ashby, 1956).
Together, the work of Bertalanffy, Wiener, and Ashby formed the core of systems theory as applied to engineering and technology. Bertalanffy’s holistic view of systems, Wiener’s exploration of feedback and control, and Ashby’s focus on self-regulation have been instrumental in shaping how engineers approach system design and optimization. These scholars emphasized the importance of considering the relationships between system components, not just their characteristics, to understand and optimize system behavior.
Systems theory under engineering and technology originated from the pioneering work of Ludwig von Bertalanffy, Norbert Wiener, and Ross Ashby. Their contributions laid the foundation for understanding complex systems as dynamic, interdependent entities. The development of systems theory has had a profound impact on how engineers design and analyze systems, emphasizing the importance of feedback, self-regulation, and holistic thinking. Today, the principles of systems theory continue to inform the design of complex systems in engineering fields such as control systems, robotics, and automation.
1.2) APPLICATION OF SYSTEMS THEORY IN ENGINEERING AND TECHNOLOGY
The application of Systems Theory in engineering and technology has significantly transformed the way complex systems are understood, designed, and managed. By focusing on the interactions between system components and their emergent properties, Systems Theory provides a comprehensive framework for engineers to address the challenges posed by intricate and dynamic systems. Its application spans multiple engineering disciplines, including control systems, robotics, network design, and automation, where understanding the behavior of a system as a whole is essential for optimizing performance and ensuring stability.
In control systems engineering, Systems Theory plays a pivotal role in the design and operation of systems that require real-time feedback to maintain desired performance. The concept of feedback loops, introduced by Norbert Wiener through his work on cybernetics, is central to the application of Systems Theory in this field. Feedback mechanisms allow a system to adjust its behavior based on the difference between its actual performance and the desired output. For instance, in temperature control systems, a thermostat continuously monitors the temperature and adjusts the heating or cooling mechanisms to maintain the set temperature. This dynamic interaction between components, guided by feedback loops, ensures the stability of the system. The application of feedback has been further advanced with the integration of modern technologies such as machine learning and artificial intelligence, enabling systems to adapt to more complex environments (Wiener, 1961).
In robotics, Systems Theory provides the foundation for designing autonomous machines that interact with their environment and make decisions based on real-time data. Robots are often required to perform tasks with high precision and adaptability, such as in manufacturing or medical procedures. Systems Theory enables engineers to model robots as integrated systems where sensors, actuators, and control algorithms work together to achieve a specific goal. The concept of self-regulation, as proposed by Ross Ashby, is particularly important in robotics, where robots must autonomously adjust their behavior to compensate for external changes, such as variations in object position or environmental conditions. This idea of adaptive control allows robots to improve their performance over time, making them more efficient and effective in various applications (Ashby, 1956).
In network design and telecommunications, Systems Theory has been used to model and optimize communication networks, ensuring the efficient transmission of data across complex infrastructures. A communication network can be seen as a system with various interconnected components, including routers, switches, and communication links. Systems Theory enables engineers to analyze how these components interact and how the system as a whole behaves under different conditions. For example, in packet-switched networks, engineers use Systems Theory to optimize routing algorithms, ensuring that data packets are transmitted efficiently while minimizing latency and packet loss. The application of feedback in network systems is also crucial in managing congestion and ensuring network stability. By continuously monitoring network traffic and adjusting routing decisions based on real-time data, engineers can ensure that the network functions optimally even as conditions change (Bertalanffy, 1968).
In automation and manufacturing, Systems Theory has been instrumental in the design of automated production lines and processes. Automation systems rely on a combination of sensors, actuators, and controllers to monitor and regulate the production process. Systems Theory helps engineers design these systems by considering how the components interact to achieve the desired output. The application of self-regulation and feedback mechanisms is particularly important in automated systems, where continuous adjustments are needed to maintain product quality and optimize production efficiency. For example, in an automated assembly line, sensors may monitor the position of components, and feedback loops will ensure that any deviations from the desired assembly process are corrected in real-time. This integration of systems thinking allows engineers to design highly efficient and reliable automated systems that can operate autonomously with minimal human intervention (Wiener, 1961).
The application of Systems Theory in engineering and technology enables engineers to design and optimize complex systems by focusing on the interactions and interdependencies of system components. Whether in control systems, robotics, network design, or automation, Systems Theory provides a holistic approach that is essential for managing dynamic, real-time systems. By emphasizing feedback, self-regulation, and adaptation, the theory continues to shape the development of modern engineering technologies that require systems to function efficiently and autonomously in complex environments.
1.3) SIGNIFICANCE OF SYSTEMS THEORY IN THE 21ST CENTURY, PARTICULARLY IN THE FIELDS OF ENGINEERING AND TECHNOLOGY
The significance of Systems Theory in the 21st century, particularly in the fields of engineering and technology, has become more pronounced as complex systems have grown in scale, intricacy, and interconnectedness. Systems Theory offers a comprehensive framework that helps engineers and technologists navigate the challenges posed by these sophisticated systems, guiding the design, optimization, and management of systems that are dynamic, adaptive, and capable of self-regulation. With the rapid advancement of technology, particularly in fields like robotics, artificial intelligence, cyber-physical systems, and the Internet of Things (IoT), the relevance of Systems Theory has never been more critical.
In modern engineering, Systems Theory is pivotal in the development of autonomous systems, such as robots and self-driving vehicles. The application of feedback loops, a key principle of cybernetics introduced by Norbert Wiener, is integral to ensuring that these systems can continuously monitor and adjust their behavior in real time. For instance, self-driving vehicles rely on sensors to gather data about their surroundings, which is then processed to make decisions on navigation, speed, and safety. Systems Theory allows engineers to model these systems holistically, ensuring that all components work in concert to create a functioning autonomous system. The ability to design systems that can adapt to unpredictable environments, adjust their behavior based on feedback, and optimize performance over time is essential for the success of technologies like autonomous vehicles and drones (Wiener, 1961).
In the field of robotics, Systems Theory is vital for understanding and designing robots that can interact with their environment, learn from their experiences, and perform tasks autonomously. The principle of self-regulation, as articulated by Ross Ashby, is particularly relevant in modern robotics, where robots need to adapt to changing conditions, such as alterations in the environment or unexpected obstacles. The significance of Systems Theory in this context lies in its ability to guide the creation of adaptive control systems, enabling robots to make decisions without direct human input. For instance, industrial robots in manufacturing environments rely on feedback systems to adjust their operations, optimizing efficiency and reducing errors. These self-regulating mechanisms are crucial for ensuring that robots perform tasks reliably, even as conditions change (Ashby, 1956).
The integration of Systems Theory is also evident in the rise of cyber-physical systems (CPS), which are systems that combine physical processes with computation and communication networks. These systems are foundational to the development of smart cities, healthcare technologies, and industrial automation. Systems Theory enables engineers to design CPS that can effectively manage the interaction between physical and digital components. For example, in smart city infrastructure, sensors embedded throughout the city collect data on traffic, energy consumption, and air quality, which is then processed by computational systems to make real-time decisions about traffic lights, energy distribution, and emergency responses. The ability to model and optimize these interconnected systems using the principles of Systems Theory ensures that cyber-physical systems can operate efficiently, adapt to changing conditions, and provide real-time responses to various challenges (Bertalanffy, 1968).
Furthermore, the growing prominence of the Internet of Things (IoT) has highlighted the importance of Systems Theory in designing and managing networks of interconnected devices. IoT systems often involve large numbers of devices communicating and interacting with each other, making it critical to understand the dynamics of the system as a whole. Systems Theory allows engineers to model these networks, optimize communication protocols, and ensure that the system can function cohesively despite the complexity of interactions between individual devices. In the context of smart homes, for example, IoT systems allow devices such as thermostats, lights, and security cameras to communicate with one another, ensuring that the home environment remains comfortable, secure, and energy-efficient. Systems Theory helps ensure that these devices work together seamlessly, maintaining stability and performance across the system (Bertalanffy, 1968).
Additionally, Systems Theory plays a key role in optimizing energy systems, particularly with the rise of renewable energy sources and the need for sustainable energy management. The transition to renewable energy sources, such as solar and wind power, requires the integration of multiple subsystems, such as energy storage, grid management, and power distribution. Systems Theory provides the tools for engineers to design these integrated systems, ensuring that energy is efficiently produced, stored, and distributed. The use of feedback systems is essential for managing fluctuations in energy supply and demand, optimizing energy use, and reducing waste. For example, smart grids use real-time data to adjust the flow of electricity based on current demand, ensuring a stable and efficient distribution of power. This application of Systems Theory is crucial for developing sustainable energy solutions in the 21st century (Wiener, 1961).
The significance of Systems Theory in the 21st century in engineering and technology lies in its ability to provide a holistic approach to understanding and designing complex, dynamic systems. As technologies continue to evolve and become more interconnected, Systems Theory remains a critical framework for optimizing performance, ensuring stability, and enabling adaptability in fields such as robotics, autonomous systems, cyber-physical systems, IoT, and energy management. The concepts of feedback, self-regulation, and interdependence are essential for navigating the challenges posed by modern technological advancements and ensuring the continued success and sustainability of these systems.
1.4) SCHOLARS’ CRITICISM OF THE SYSTEMS THEORY UNDER ENGINEERING
Systems Theory in engineering and technology, while highly influential, has faced various criticisms from scholars regarding its application, comprehensiveness, and limitations in addressing real-world complexities. Despite its foundational contribution to the understanding of complex systems, several critical points have been raised regarding its scope and utility in modern engineering and technological practice.
One significant criticism of Systems Theory is its tendency to oversimplify the complexity of real-world systems. Scholars have pointed out that while Systems Theory emphasizes the importance of a holistic perspective, it often fails to account for the intricate, emergent behaviors that can arise in complex systems. For instance, Checkland (1981) argued that the theory’s emphasis on a systems approach can sometimes obscure the complexity of individual components, which may interact in unpredictable ways. This reductionist view, where the behavior of a system is assumed to be entirely determined by the interactions of its parts, is often insufficient to capture the full complexity of systems that involve non-linear interactions or those affected by chaotic dynamics. Systems Theory assumes that by understanding the system as a whole, one can predict and control the behaviors of its parts, which does not always hold in practice, especially in systems involving human factors or environmental unpredictability.
Another critique comes from the limited attention given to human and social factors in the design of technical systems. While Systems Theory is adept at modeling mechanical and technological components, it often overlooks the crucial role of human agency and social dynamics in system performance. Ulrich and Reynolds (2010) noted that the theory frequently neglects the complexities introduced by human behavior, decision-making, and organizational factors, which are essential for understanding how systems function in the real world. In fields like industrial automation and healthcare systems, for example, human operators are integral to the system’s effectiveness, and neglecting this aspect may lead to inefficient or unsafe designs. The human and social elements must be integrated into the system’s design for it to function optimally, a concern often overlooked by traditional Systems Theory.
Furthermore, critics have pointed out that Systems Theory tends to neglect ethical, environmental, and societal considerations, which have become increasingly important in modern technological development. Meadows (2008) observed that while Systems Theory is concerned with system optimization and efficiency, it often ignores broader ethical implications, such as the impact of technology on privacy, security, and the environment. As technologies such as the Internet of Things (IoT) and autonomous systems become more integrated into daily life, the ethical and environmental consequences of these systems require closer attention. Systems Theory, with its focus on technical and functional aspects, may fail to address issues like the potential for surveillance or the environmental footprint of technological systems, which are critical concerns in today’s technological landscape.
Additionally, the application of Systems Theory to non-linear and highly dynamic systems has been criticized. Scholars such as Gleick (1987) argued that Systems Theory, which is grounded in concepts like feedback loops and equilibrium, struggles to model systems that exhibit chaotic behavior or are characterized by unpredictable, non-linear interactions. In fields such as climate modeling or financial markets, small changes can lead to disproportionately large effects, and the systems’ behavior may not be easily predictable or controllable. The complexity of such systems challenges the assumptions of Systems Theory, which tends to focus on achieving stability and balance. This gap in the theory has led to the development of alternative frameworks, such as chaos theory and complex systems theory, which provide better tools for understanding these unpredictable, dynamic systems.
Finally, the theoretical nature of Systems Theory has been criticized for being too abstract and disconnected from practical, real-world applications. While the theory provides a broad conceptual framework for understanding systems, its generality can make it difficult to apply directly to specific engineering problems. Meadows (2008) argued that the general nature of Systems Theory often fails to provide the detailed methodologies required for solving concrete engineering challenges. The theory’s focus on high-level concepts such as feedback, interdependence, and system boundaries may not always translate into actionable guidance for engineers working on complex technological problems. For practical applications, engineers often need more specific tools and methods that can address particular challenges in system design and optimization, something that Systems Theory is often criticized for lacking.
In conclusion, although Systems Theory has made significant contributions to engineering and technology, it faces several criticisms. These include its oversimplification of complex systems, neglect of human and social factors, failure to address ethical and environmental concerns, limitations in modeling non-linear and chaotic systems, and its abstract nature, which may hinder practical application in specific engineering contexts. These criticisms highlight the need for a more nuanced and integrated approach that incorporates the diverse challenges and considerations of modern technological and engineering systems.
1.5) SUMMARY AND CONCLUSION
The application of Systems Theory in engineering and technology has been instrumental in fields such as automation, control systems, communications, and product design. It has helped engineers develop more efficient systems, ensure better coordination between different components, and manage complexity in large-scale systems. The theory’s emphasis on feedback loops, system boundaries, and interconnections has proven valuable in understanding dynamic systems that operate in changing environments.
However, while Systems Theory has had a profound impact, its application is not without limitations. One of the key challenges is that it can oversimplify complex real-world systems, especially when these systems involve unpredictable behaviors or interactions between various stakeholders. Additionally, Systems Theory often overlooks human and social factors, which are crucial in many engineering and technological applications. Critics argue that its focus on technical and functional optimization may neglect ethical, environmental, and societal considerations that are increasingly important in the modern technological landscape.
Despite these criticisms, Systems Theory remains an essential tool in engineering and technology, providing a foundational understanding of how systems function and interact. For it to remain relevant in the 21st century, however, it must adapt to address the growing complexity and unpredictability of modern systems, as well as integrate social, ethical, and environmental factors into its frameworks. This will ensure that Systems Theory continues to provide valuable insights and practical solutions in the ever-evolving fields of engineering and technology.
In conclusion, while Systems Theory has made significant contributions to the development of engineering and technology, it must evolve to address its limitations. Incorporating new methodologies that account for non-linear dynamics, human factors, and broader societal impacts will enhance its applicability and ensure that it remains a critical tool in the design, management, and optimization of complex systems in the future. By doing so, Systems Theory can continue to guide engineers and technologists in creating more effective, sustainable, and ethically sound systems.